Sulfate III: Its All About Blood Flow - Why Sulfation Is Prioritised
- emoverton
- Jul 21, 2018
- 11 min read
In the first article of this series, I introduced the reader to the sulfation pathway and provided a basic overview of how the body obtains and utilises sulfur from different sources in the diet. The second article focused on synthesising various concepts surrounding the "living matrix" system and endogenous structured water, and further highlighted some of the ways in which this may be important for human physiology
In this article, I am going to connect the content of the previous two articles together so that hopefully you can begin to appreciate the role sulfate plays in maintaining life. The majority of the work presented here is based on the research of Dr Stephanie Seneff and colleagues. The original content and ideas are not my own, and this piece is simply an overview of my reading around the subject.
I am going to focus on the roles of cholesterol sulfate in red blood cells and sulfated glycosaminoglycans within the capillary wall, and hopefully shed some light on how the biophysical properties of these two materials help to facilitate healthy blood flow through the microvasculature.
Introduction
The sulfation pathway plays many different roles in the human body. Sulfate is utilised as a way of detoxifying harmful toxins and waste products of metabolism. Sulfate is also used to synthesise large extracellular macromolecules such as mucopolysaccharides. In fact, the body likes to sulfate a whole host of endogenous chemicals:
Sulfated steroids (including cholesterol and DHEA)
Sulfated phenols (including estradiol, capsaicin, salycilates, and neurotransmitters such as dopamine and serotonin,)
Sulfated tyrosine
Sulfated bilirubin
Sulfolipids
It turns out that sulfate can be attached to many different macromolecules. Not that much sulfate is found floating freely in the blood, however. As we will see, having too much free sulfate in the blood could lead to potentially fatal consequences.
Sulfate as a Kosmotrope
Sulfate is classified as a powerful kosmotropic ion. Without going into detail, the "Hofmeister series" is a classification of different ions based on their ability to interact with and organise proteins in a solution. These effects can also be seen in an ion's interaction with water. "Chaotropes" are ions which induce disorder in water (promote bulk water) whereas "kosmotropes" are ions which are known to "organise" water into an exclusion zone gel. Since sulfate is a kosmotrope, it has a strong gelling effect on water. Hence, too much un-bound sulfate flowing through the blood could actually lead to clot formation, and ultimately death of the host.
One of the main ways to bypass this issue and safely transport sulfate is via attaching the sulfate to a carrier molecule. A suitable carrier molecule is cholesterol. Sulfation of cholesterol yields cholesterol sulfate.
Cholesterol is mostly a non-polar fatty substance which is not soluble in blood, so it needs to be transported via lipoproteins (think LDL and HDL). However, cholesterol sulfate isampiphillic, which means that it is both water AND fat soluble. Cholesterol sulfate travelling through the blood is a handy way to deliver cholesterol to the places that it needs to get to without having to rely on other transport methods. Furthermore, transporting sulfate in this way avoids excessively thickening the blood.
Cholesterol sulfate is found in large quantities on the membranes on red blood cells. Here, it plays crucial roles in maintaining membrane integrity and providing the cells with properties that enable them to flow through the vessels.
Dr Stephanie Seneff and colleagues have written about cholesterol sulfate extensively. In one paper titled "A novel hypothesis for atherosclerosis as a cholesterol sulfate deficiency syndrome" the authors theorise that the synthesis of cholesterol sulfate occurs when the skin and surface capillaries are exposed to direct sunlight. The key player here is an enzyme called endothelial nitric oxide synthase (eNOS). Providing there is sufficient dietary supply, eNOS catalyses a reaction which combines sulfur and cholesterol to produce cholesterol sulfate. Newly synthesised cholesterol sulfate can then be shipped around the body via the red blood cells to distribute sulfate to the cells that line the capillary.

Sunlight and sulfation of cholesterol. Robert M. Davidson and Stephanie Seneff, “The Initial Common Pathway of Inflammation, Disease, and Sudden Death,” Entropy 2012, 14, 1399-1442.
Where is sulfate needed?
Sulfated hydrophillic projections decorate the exterior of every cell. Embedded within and attached to the membrane of each cell are various types of sulfated molecules which include cholesterol sulfate, heparan sulfate proteoglycans, and other sulfated glycosaminoglycans.
The sulfated glycosaminoglycans (sGAGS) such as chondroitin, haparan, dermatan, and keratin sulfate are critical components of the extracellular matrix. SGAGs also serve many other functions, from improving cell metabolism to DNA and RNA synthesis, clearing lipids from the blood, and accelerating healing and regeneration.
The sGAGs are utilised to form proteoglycans, a special type of glycoprotein consisting of a core protein with lots of sGAGs attached. The sGAG chains are spread out adjacent to one another, forming a "brush-like" structure. The presence of carboxylate and sulfate groups on the sGAG molecule endows it with an extremely dense negative electrical charge. Chains of sGAGs make up a significant portion of the extracellular space, and because of their negative charges, they repel one another. This creates a domain of negative charge around the entire cell. These external hydrophillic sulfate anions are suitable for building thick layers of gel-like exclusion zone water at the interface with each proteoglycan chain.
The shell of water around cells appears to provide significant protection. The membrane of each cell houses a variety of vulnerable molecules (like polyunsaturated fatty acids) which are susceptible to damage when exposed to oxidative influences. A thick hydration shell of EZ water completely surrounding its surfaces can effectively exclude solutes - which applies to practically anything other than electrons - to act as a protective layer around the cell. We can see that it is possible that the EZ hydration shell formed on the outside of the cell may effectively create a protective barrier between the cell membrane and the surrounding environment. This could theoretically prevent damage from occurring as a result of contact with the oxidative conditions in the external environment (such as the blood)..

Wikimedia Commons
The Glycocalyx
Certain cells lining the surfaces within the body are called "epithelial cells". They can be found on the surface of the tissue which lines the lungs, the mouth, the throat, the intestine, and the vascular system etc. The cells lining the blood vessels have a thick, fuzzy coating on their external membranes called the endothelial glycocalyx layer, which is rich in sGAG projections - particularly heparan sulfate proteoglycans.

Sennef, S. (2015). Cholesterol Sulfate, Electricity, and the Vasculature.

Reitsma, S., Slaaf, D., Vink, H., van Zandvoort, M. and oude Egbrink, M. (2007). The endothelial glycocalyx: composition, functions, and visualization. Pflügers Archiv - European Journal of Physiology, 454(3), pp.345-359.
Due to the abundance of sGAGs and sulfate proteoglycan molecules ebedded within the glycocalyx layer, this area of cells becomes a likely candidate for building structured EZ water. It is also a vast domain of negative charge (as the diagram demonstrates below)

Seneff, S., Davidson, R., Lauritzen, A., Samsel, A. and Wainwright, G. (2015). A novel hypothesis for atherosclerosis as a cholesterol sulfate deficiency syndrome. Theoretical Biology and Medical Modelling, 12(1).
Blood flow
According to Gerald Pollack, much of the water inside the cell is in close proximity to hydrophillic surfaces and is therefore likely structured. Likewise, this is also applicable to the extracellular water.
One of the exceptions to this is flowing blood. Fluidity of the blood needs to be maintained so that it can travel freely throughout the vascular system to rapidly deliver oxygen and nutrients to tissue, and then subsequently remove waste materials and toxic metabolites.
The blood is filled with negatively charged particles. Charge carriers include: platelets, proteins like albumin and globulins, fatty particles like LDL and HDL, and also fragments such as heparan sulfate. The dense concentration of cholesterol sulfate and sialyc acid on the membrane of red blood cells (RBCs) provide the cells with a negative charge. This negative charge produces a repulsion between individuals cells which prevents aggregation and unnecessary clot formation. This is due to something called the "zeta potential". The zeta potential is a measure of the degree of repulsion between similarly charged particles in suspension, which in this context is the contents of the blood.
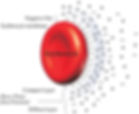
Blood cells need to maintain a high zeta potential (a highly repulsive force against other cells) to stop them from clumping together and forming aggregates. Low zeta potential means that cells are more likely to form blood clots, whereas high zeta potential reflects healthy blood. The zeta potential of the blood cell is largely determined by the cell's quantity of sulfate. As we saw above, the glycocalyx is also has a strong negative charge.
Since similar charges repel one another, the resulting electrostatic repulsion between the capillary wall and red blood cells keeps the cells from becoming lodged, or "stuck". The structured water blanket built at the interface of the glycocalyx also provides a smooth, slick layer over which the blood cells can freely pass.

Sennef, S. (2015). Cholesterol Sulfate, Electricity, and the Vasculature.
Accordingly, one of the roles of cholesterol sulfate is to deliver sulfate to the glycocalyx layer lining the capillary. Red blood cells do this by "shedding" cholesterol sulfate to re-supply the vascular surfaces with additional negative charge as they enter from the artery and travels toward the vein (left to right side of the diagram below). Once red blood cells have dropped off the cholesterol sulfate, they become more positively charged.

Sennef, S. (2015). Cholesterol Sulfate, Electricity, and the Vasculature.
This shedding action leads to an unequal distribution of charge across the capillary (or "voltage drop"), where the artery is more negatively charged and the venous side is more positively charged. The voltage difference is enhanced when carbon dioxide is picked up by the blood. This distribution of charge effectively creates a battery-type system which helps to propel or "pull" the blood cells through the tight lumen of the capillaries.
Electrokinetic Vascular Streaming Potential
The pumping of the heart creates pressure which is used to push blood through the vessels. The high zeta potential engendered by the dense negative charge on the surface of red blood cells, coupled with the flow of these charged cells through the blood, generates what is referred to as the "electrokinetic vascular streaming potential" (EVSP). According to the authors, a high EVSP stimulates the synthesis of nitric oxide via eNOS on the venule side of the capillary.
In other words, the cells lining the capillary respond to the electromagnetic field (generated by flowing red blood cells) by synthesising nitric oxide, an endogenous vasodilator responsible for relaxing the blood vessels. This action promotes blood flow from the capillary toward to vein.

Seneff, S., Davidson, R., Lauritzen, A., Samsel, A. and Wainwright, G. (2015). A novel hypothesis for atherosclerosis as a cholesterol sulfate deficiency syndrome. Theoretical Biology and Medical Modelling, 12(1).
"Electrostatic repulsion prevents RBCs from adhering to the capillary wall, and the encasement of the glycocalyx within structured water, formed in response to the kosmotropic properties of sulfate, provides a smooth surface further protecting from adhesion.The RBCs are propelled toward the venous end of the capillary by the electromagneticfield induced by the voltage drop across the capillary. The RBCs themselves act as moving charged particles that create a circular magnetic force around the capillary wall. This force induces nitric oxide release from the endothelial cells, which promotes vascular relaxation and increases flow." (Seneff et al, 2015)
What are some of the real-life implications of this?
The viscosity (thickness) of the blood is determined by the degree to which suspended cells and particles repel one another (zeta potential). If there is a high zeta potential, the blood is stable and is able to flow freely. On the other hand, a low zeta potential leads to aggregation and clot formation. The zeta potential is determined by the amount of negatively charged sulfates present on the membrane of the cell.
Likewise, the smooth glycocalyx layer lining the endothelium of the capillary is also a crucial component of this system. The relative smoothness of the glycocalyx surface depends on its ability to build a blanket of structured water at the interface. Again, this process is also reliant on sulfate.
It is worth noting here that the rate-limiting step in the production of sulfated glycosaminoglycans is the availability of 3'-Phosphoadenosine 5'-phosphosulfate (PAPS) - the ACTIVATED form of sulfate.
In simple terms: if there is low sulfate availability, there will be less sulfated glycosaminoglycan synthesis, period! Less sulfated glycosaminoglycans means lower ability to structure water in the capillary and less negative chagre, which spells BIG TROUBLE for the cardiovascular system.
The body will always prioritise blood flow
Lets take a step back to attempt to comprehend the significance of the above set of information. Blood flow is perhaps singularly the most important function to maintain survival. When the blood flow stops, we die. It is really that simple. This means that the body will always prioritise blood flow.
The work of Dr Seneff and co has demonstrated that sulfate is essential for maintaining the blood flow. Not only does it provide a dense blanket of EZ water and negative charge to the capillary glycocalyx, it is also embedded into the membrane of the blood cells themselves to maintain structure and to create a repulsive force which prevents clotting.
So what happens when the body becomes depleted of sulfate?
Disrupted sulfur metabolism can essentially lead to a situation where the body is deprived of sulfate. A detailed summary of the various ways by which sulfur metabolism can get screwed up is way beyond the scope of this article. Dr Sennef and colleagues have already done an extraordinary job at explicating the details of this already, so for the reader who is interested, I would recommend starting with the following papers:
A novel hypothesis for atherosclerosis as a cholesterol sulfate deficiency syndrome
Is Cholesterol Sulfate Deficiency a Common Factor in Preeclampsia, Autism, and Pernicious Anemia?
Aluminum-Induced Entropy in Biological Systems: Implications for Neurological Disease.
Biological Water Dynamics and Entropy: A Biophysical Origin of Cancer and Other Diseases
Glyphosate, pathways to modern diseases II: Celiac sprue and gluten intolerance
Glyphosate, pathways to modern diseases IV: cancer and related pathologies
Glyphosate pathways to modern diseases V: Amino acid analogue of glycine indiverse proteins
Glyphosate pathways to modern diseases VI: Prions, amyloidoses and autoimmune neurological diseases
In short, multiple environmental factors likely contribute to poor sulfur metabolism. However, the main culprits appear to be glyphosate (round-up ready herbicide), aluminium, mercury, and other heavy metals. Disrupted enzymes, deficient cofactors, lack of transporters, and dysbiosis are each part of the picture.
In the scenario of sulfate deficiency (which I believe is quite common nowadays), it would appear that the body becomes reliant on a set of "backup mechanisms" to recover and replenish depleted sulfate. Seneff's hypothesis proposes that many of the so-called pathological "diseases" we see today may in fact be intelligent attempts made by the body to replenish sulfate.
Because sulfate is so fundamentally important for maintaining basic functions of the cell, we can see why it would probably be prioritised to ensure the short-term survival of an organism. Without it, death would rapidly ensue.
Hence, with the aim of replenishing this fundamental element, the body seemingly behaves in ways which appear counter-productive when viewed from a narrow perspective. Sennef provides several examples of how "pathological" events may actually be beneficial adaptations:
Atherosclerotic plaque: An atheroma located in the artery supplying the heart is a deposit of fatty substances and cholesterol. According to Seneff's theory, the fatty deposit is designed to 'pick up' any available sulfate to synthesise cholesterol sulfate, and is conveniently located so that it can supply the cholesterol sulfate to a deficient heart.
Heart attack: Heart attack is characterised by a significant increase in superoxide production (a radical capable of producing oxidative damage). A massive burst of taurine is released from the heart during inchemia. Taurine is an amino acid with a high sulfur content. Sennef proposes that the superoxide yield could theoretically be utilised to oxidise taurine's sulfur into sulfate, thereby replenishing the supply.
Elevated Homocysteine: High homocysteine is generally assumed to be a "bad" biomarker, and the typical way of treating this is to target the methylation pathway (for example, supplementing with methyl B12, Folate, B6 etc). Homocysteine is also an amino acid which contains sulfur, however. Homocysteine thiolactone can be oxidised to sulfate via a reaction with superoxide, using vitamin C and vitamin A as cofactors. Seneff argues that high homocysteine may therefore be a method of supplying sulfate to the vasculature.
Chlamydia Pneumoniae infection: Chlamydia Pneumoniae is an microbial pathogen which has been found to colonise the atheromatic plaque in atherosclerosis, This has led some researchers to look at the infection as a potential cause of the plaque buildup. Interestingly, this specific microbe is able to synthesise a form of heparan sulfate. Seneff hypothesises that this infection is intelligently selected for as a way to supply sulfate to the atheroma.
For more information, you can listen to the podcast where we interviewed Dr Seneff HERE!
Next up: Exploring SIBO/Dysbiosis as a way to replenish sulfate....
References
Davidson, R. and Seneff, S. (2012). The Initial Common Pathway of Inflammation, Disease, and Sudden Death. Entropy, 14(8), pp.1399-1442.
Fernandes, H., Cesar, C. and Barjas-Castro, M. (2011). Electrical properties of the red blood cell membrane and immunohematological investigation. Revista Brasileira de Hematologia e Hemoterapia, 33(4), pp.297-301.
Reitsma, S., Slaaf, D., Vink, H., van Zandvoort, M. and oude Egbrink, M. (2007). The endothelial glycocalyx: composition, functions, and visualization. Pflügers Archiv - European Journal of Physiology, 454(3), pp.345-359.
Seneff, S., Davidson, R., Lauritzen, A., Samsel, A. and Wainwright, G. (2015). A novel hypothesis for atherosclerosis as a cholesterol sulfate deficiency syndrome. Theoretical Biology and Medical Modelling, 12(1).
Seneff, S. (2015). Cholesterol Sulfate, Electricity, and the Vasculature.